Rivers, by nature, are dynamic and complex. When river systems are connected to their floodplains, they can breathe and recover from natural disturbances.
Research fish biologist Rebecca Flitcroft remembers standing on the banks of the lower South Fork McKenzie River gazing across the valley at recently completed floodplain restoration work. Mosaic-like features had been dug on both sides of the river. Logs stuck out of sand and water. Shallow log piles lay where the main channel had previously been, causing the river to overflow and spread out.
“The messiness of that post-restoration environment was confusing to look at,” says Flitcroft, with the USDA Forest Service Pacific Northwest (PNW) Research Station. “For most people, tidy looks best, but that may not be true for aquatic ecosystems.”
This was not the river after a flood or landslide. The digging, the log piling, and the addition of boulders and gravel was a deliberate attempt to rehabilitate the floodplain of the South Fork McKenzie River. The end goals were a healthy habitat for native fish and other river life, improved water quality, and reestablishing the river system’s natural flow. This chaotic-looking method may seem counterintuitive, but projects like this reflect the hypothesis that messy rivers are healthy rivers.
Various watershed councils and Forest Service units have been applying floodplain reset restoration methods such as these in the Pacific Northwest for the past 10 years. Wanting empirical data to assess the effectiveness of the methods, Forest Service staff from the Pacific Northwest Region asked scientists with the PNW Research Station such as Flitcroft, other federal agencies, and universities to identify metrics and assess the efficacy of this kind of floodplain rehabilitation around the South Fork McKenzie River and other sites in Oregon.
“We need to understand the short- and long-term effects of floodplain restoration for aquatic ecosystems, which includes the fish, bugs, and other organisms that occupy resilient habitats,” says Flitcroft.
The projects seek to restore dynamic sediment and water conditions to floodplains, with the goal of improving the health of the river systems that they were once connected to. “All this research and experimental restoration effort reflects the urgency to understand how to recover aquatic ecosystems,” says Flitcroft. “We were asked to do a synthesis of what we know up until now. What does this form of floodplain restoration do?”
Floodplain reset
Floodplains are crucial to providing calm, shallow waters for aquatic life. Fish thrive in streams with side channels that support a smorgasbord of aquatic life, as well as logs that create pools of deeper, slower water. Fish also benefit from streams with overhanging branches that provide shade. Stream substrate is also important. Native salmon need gravel that’s half an inch to 3 inches in diameter to spawn. Large wood deposits also become homes for small land animals and birds. And as the wood decays, it nourishes the floodplain soil, supporting vegetation.
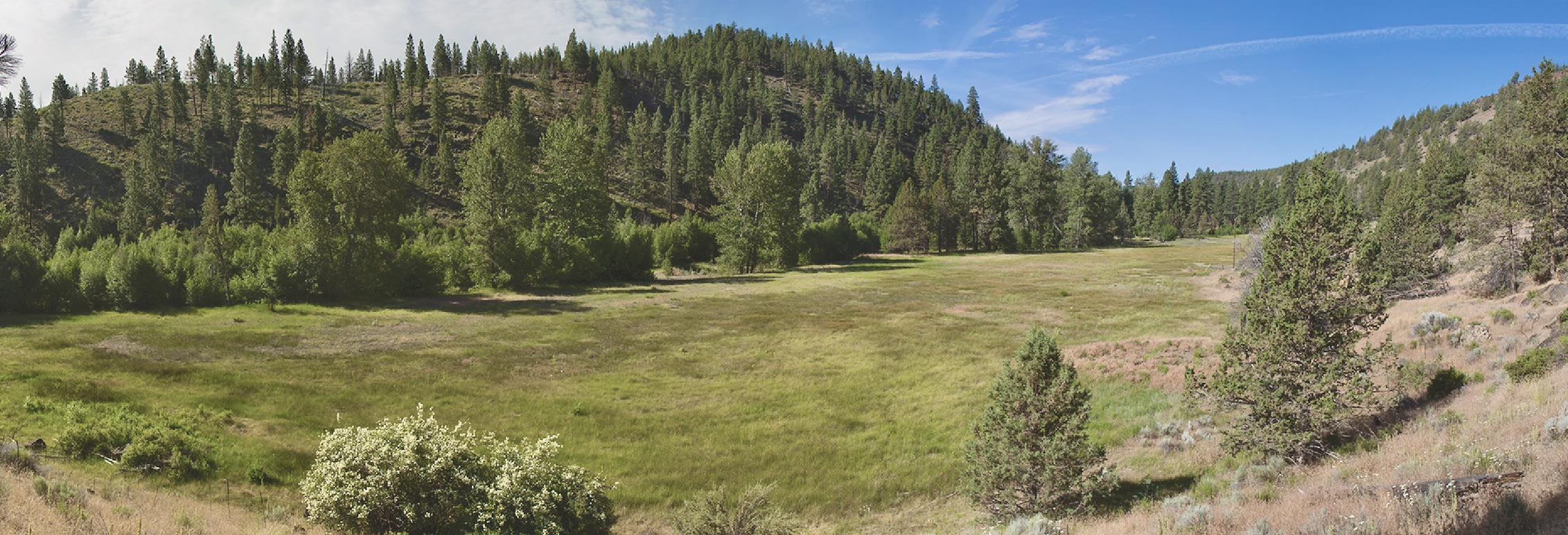
To this end, restoration teams across Oregon have been dislodging levees, allowing their contents to spill into deep river channels. They have deposited wood, large boulders, and gravel sediments into rivers to raise channel beds and inundate valley floors with water. The process reconnects rivers to their natural floodplains, allowing the river to rework sediments into complex mosaics of habitat.
The method is sometimes called “Stage 0 restoration” because it aims to bring the floodplain back to its condition before EuroAmerican settlers constructed dams, dikes, levees, and ditches that forced rivers and streams into deep, fast-flowing, single-thread channels. The term “Stage 0” comes from the work of Brian Cluer and Colin Thorne in 2014, which introduced a new conceptual stage in channel evolution meant to represent the starting point.
Flitcroft prefers to call it “floodplain reset.” “We generally don’t know what a pre-settlement condition looks like,” she says. “Natural processes like landslides may have filled channels in ways similar to a floodplain reset restoration, and we’re interested in understanding how aquatic ecosystems respond to this type of disturbance.”
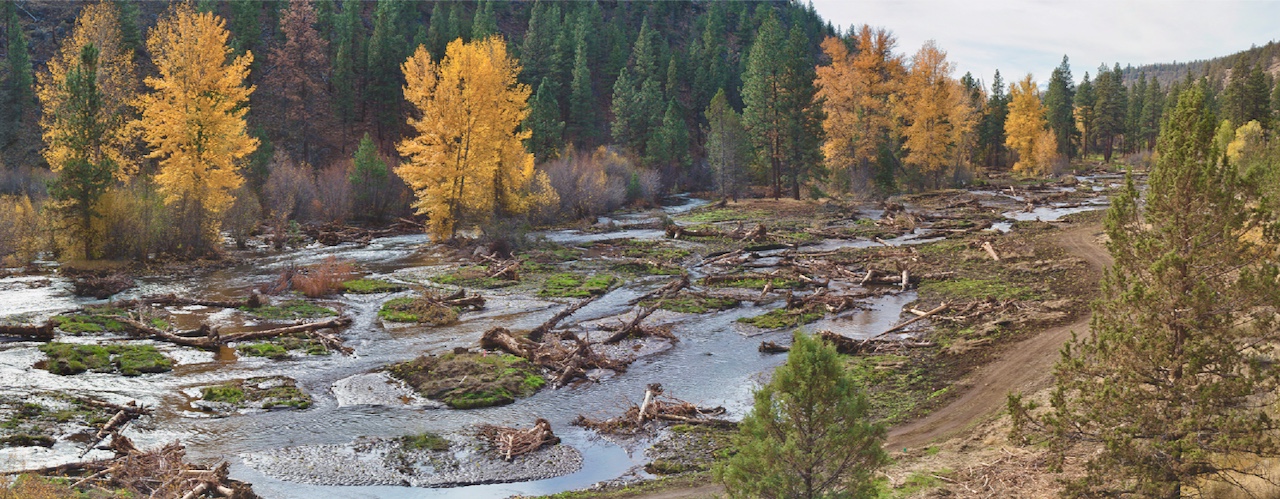
Coming together
Watershed councils, Forest Service scientists, and teams of other fish biologists and hydrologists have experimented with extensive floodplain restoration at multiple sites throughout Oregon. This includes large and small rivers in Douglas fir-dominated forests of western Oregon and high-elevation ponderosa pine forests and meadows of eastern Oregon. Restoration practitioners monitored the sites to evaluate the effect of the restoration using different metrics and methods, depending on the interest of local partners and practitioners. A large and diverse group of collaborators collected the data, including nonprofit organizations, restoration companies, university researchers, and Forest Service and other federal agency staff.
As interest in a comprehensive understanding of floodplain reset restoration actions developed, it became clear that the projects needed a holistic definition of this term to frame ongoing monitoring and specific research projects. To that end, Flitcroft and other researchers held workshops for the many groups involved in rehabilitating the river valleys.
“The workshops were an opportunity to bring together the community of practitioners of floodplain reset restoration who collectively represented a cohesive understanding of this method but had not actually put words to paper to define this approach so it could be described consistently to others,” Flitcroft says.
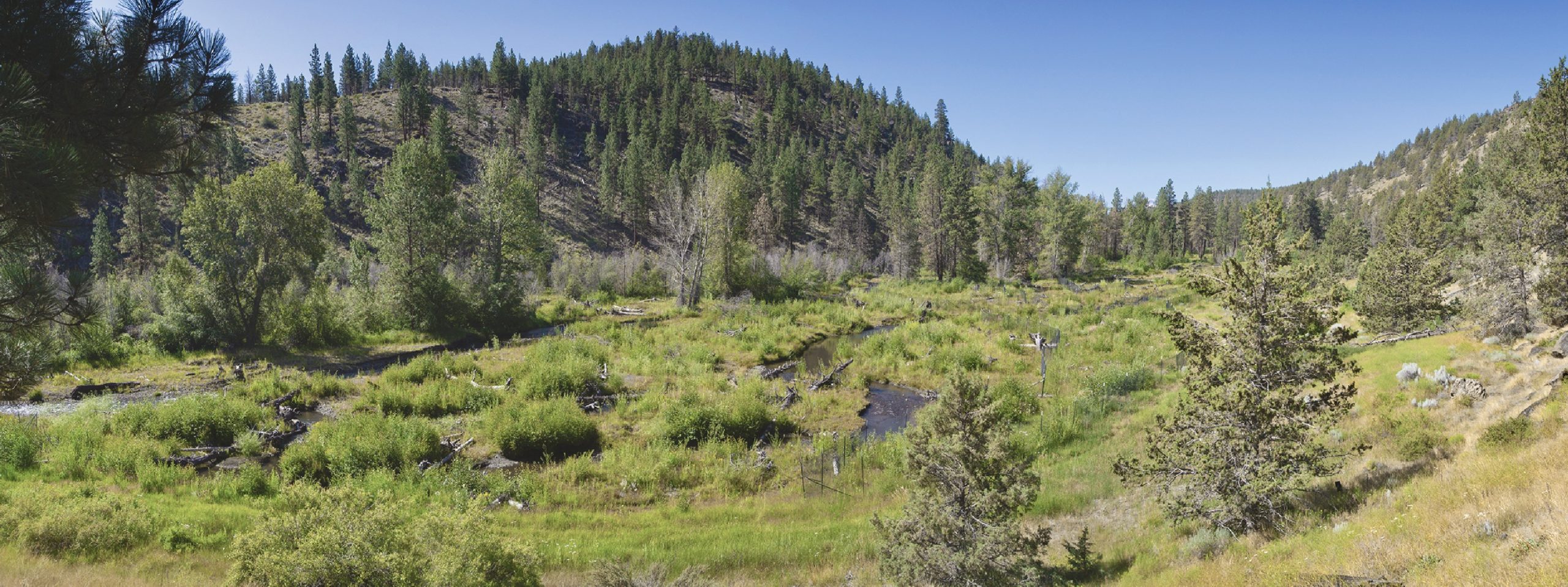
The workshops with practitioners to define floodplain reset restoration (or restoration to a Stage 0 condition) were successful, leading to a synthesis of existing monitoring data and a framework for more specific projects that used new methods and new data sources. For example, data collected via drones holds great potential for valley-scale rehabilitation efforts.
“The projects were a good opportunity to test and develop methods for a new source of data—unoccupied aerial systems, or drones,” says Lauren Mork, who manages the monitoring program for the Upper Deschutes Watershed Council.
“When you introduce all of that complexity and think about your streams as moving across the valley floor, occupying a larger proportion of the valley floor that is now scattered with large wood and supporting abundant riparian vegetation, that’s where the benefit of drones comes in,” Mork says. “In the first 3 to 5 years after restoration, you have a better opportunity to detect water, map inundated areas, map wood, and map the vegetation with multispectral imaging.” If done by humans, this kind of data collection could be logistically challenging, expensive, and even dangerous.
Early effects
After rehabilitation, the areas with surface water were larger, water flowed more slowly, and sediment texture was finer. The rivers and new streams were also rougher because of the large wood that had been placed in the channels.
“This is what we’re seeing in the first few years immediately after this type of restoration,” Flitcroft says. “And in many regards, it mimics a debris flow or a large natural landscape-scale displacement that happens to a river.”
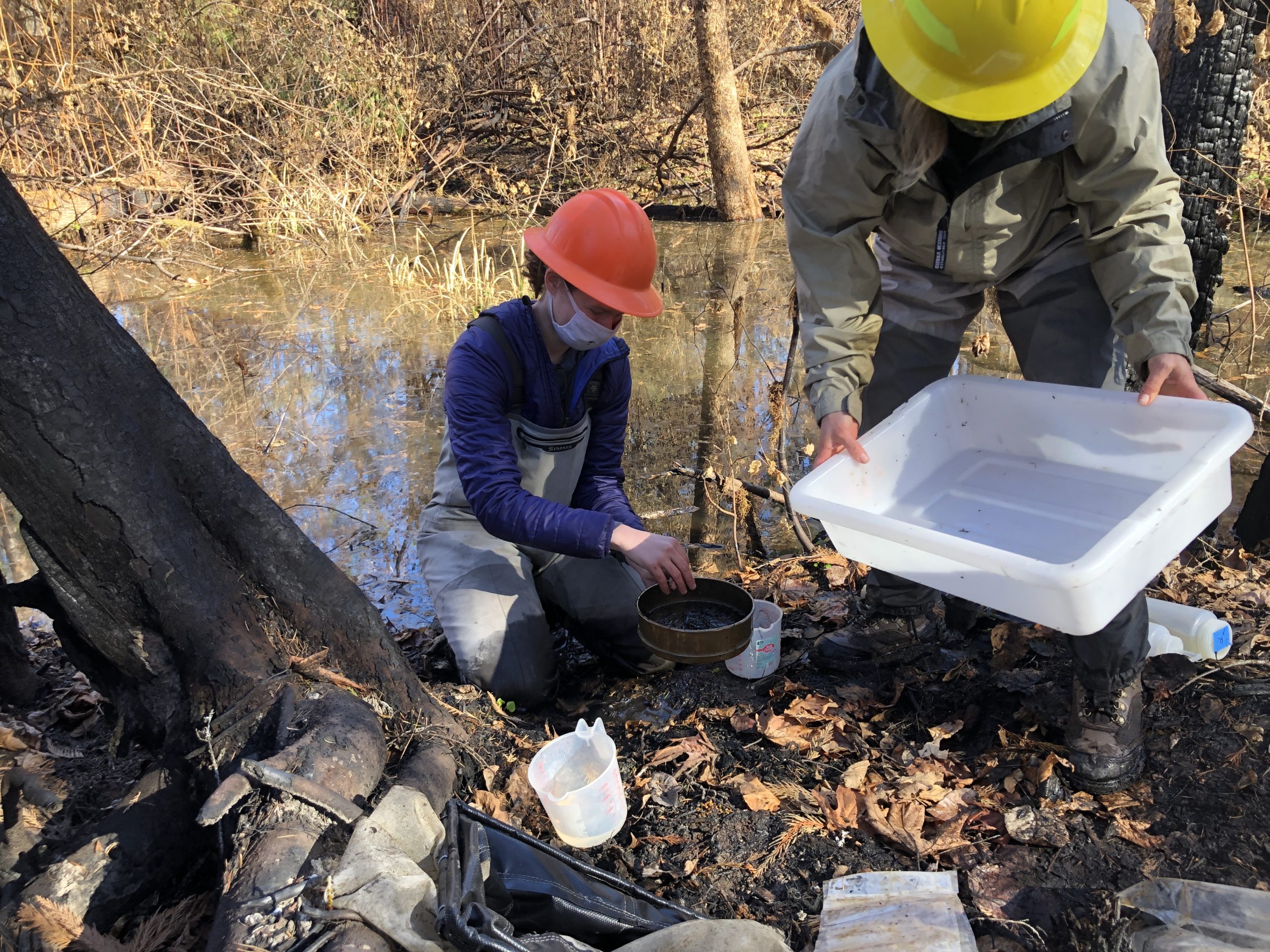
This means that the different restoration groups achieved their goals of increasing areas covered by slow-flowing water, reconnecting surface water and ground water, expanding rivers and streams, and increasing the river and floodplain’s physical complexity, or messiness—at least in the short term.
The researchers also analyzed water temperatures before and after restoration at different sites. They found varied responses in the thermal conditions. For example, at the South Fork McKenzie River site, discharge from the Couger Dam upstream complicated matters, but they did find an increase in the daily maximum water temperature the first few years after restoration. The researchers also noticed distinct areas of warmer and colder water, contributing another aspect to habitat diversity.
At restoration sites where researchers collected data on the diversity and abundance of aquatic macroinvertebrates and vertebrates, analysis revealed initial decreases immediately after rehabilitation. However, within 2 years, diversity of aquatic macroinvertebrates exceeded pre-restoration numbers, and the composition of macroinvertebrate communities became more complex further upstream compared to what they had been before restoration. Thus, as a mosaic of habitats became available, a wider array of species moved in.
However, the biomass—the total quantity, or in this case weight, of organisms per square meter—declined. This reflected a transition from large aquatic macroinvertebrates, such as juga snails, to small, quick-reproducing species, such as midges.
This decline in biomass—a product of fewer larger organisms but more smaller ones— was offset by the large area of inundation. Increases in area inundated with flowing water resulted in an overall increase in available aquatic habitat, and in the total quantity of aquatic life across the entire restoration area.
Researchers look at bottom-dwelling macroinvertebrates, such as stonefly larvae and crayfish, as indicators of river system productivity; thus, more biomass can mean higher productivity. “If you look at the scale of the whole project, the amount of biomass in the whole project area is higher,” explains Flitcroft. “But at that square-meter scale, it’s lower. There’s just so much more space now that you have more productivity than you did before. It’s just spread out.”
The researchers also looked at the interaction between floodplain connection and wildfire, after the 2020 Holiday Farm Fire burned more than 230 square miles of forest in the McKenzie River basin, including the restoration site. The study found that floodplain reset—particularly floodplain re-wetting and bringing back floodplain habitat mosaics—can reduce wildfire intensity and make burns less uniform. These could— in theory—increase a site’s resilience to climate change and enhance biodiversity.
Not for every location
Flitcroft acknowledges that floodplain resets are not a fit for every landscape. Because it encourages a historical floodplain to flood, it’s not best for areas where a lot of people live downstream or when infrastructure, such as large roads, are next to the river corridor.
She notes that the different sites responded to restoration differently and with variable timelines. “There’s still so much more we need to learn,” she says. “We have made a start at understanding where some of the variation is, and in the types of responses we might expect to see.”
William Brignon, the regional salmon recovery liaison for the Forest Service’s Pacific Northwest Region, is developing a monitoring strategy for restoration projects that includes floodplain reset. He has been involved in acquiring the permits that the National Forest System requires to do floodplain restoration.
“Our practitioners are out collecting data on a yearly basis, answering the questions that are of interest to them every year, which is critical. But at the same time, we want to make sure those data can be analyzed within and across sites to learn about the restoration technique as a whole,” Brignon says. He’s working on a plan that coordinates the use of the latest technology to make monitoring a little easier for everyone.
Brignon, Flitcroft, and their colleagues plan to monitor water depth and the contour of riverbeds by using LiDAR (light detection and ranging) data, which is typically collected via planes or helicopters. They also plan to map surface-water temperatures by using forward looking infrared, or FLIR, technology which can be collected via drones.
“We want to squeeze as many metrics out of our monitoring plan with those flights so that we can then give the data to our practitioners so they can focus their efforts on metrics that require a boots-on-the-ground approach,” Brignon says. “We’re also going to feed that back into developing the framework for the monitoring plan.” The goal is to pool all the monitoring data every 3, 5, or 10 years for analysis to learn over time and build the foundation for adaptive resource management.
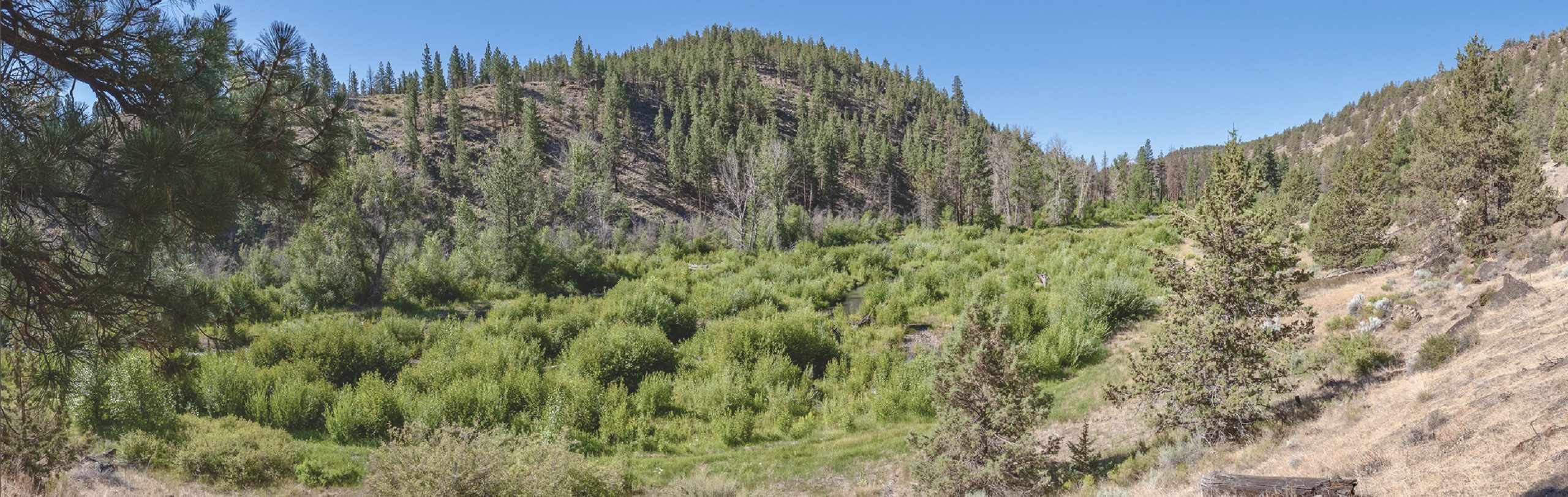
The completed research provides a foundation for future work. Developing monitoring metrics, such as those described by Brignon, helps establish new standardized data collection tools to describe the physical characteristics of floodplains both before and after restoration. Some of the existing datasets have also been archived with the Oregon Department of Environmental Quality, making data easily accessible. The results can inform conservation planning for the siting and implementation of floodplain reset rehabilitation across a diversity of ecoregions, elevations, and river sizes.
Past and present monitoring efforts will also help researchers and restoration practitioners better understand how restoration interacts with natural disturbances, such as floods, wildfires, or mudslides, in rivers systems and on floodplains.
“Many of us are much more comfortable with things being stable rather than focusing on things being resilient,” Flitcroft says. “That really works well for people. But it doesn’t work well for ecosystems, particularly ecosystems that have a history of being really dynamic.”
Rivers, by nature, are dynamic and complex. When river systems are connected to their floodplains, they can breathe and recover from natural disturbances.
Originally published in Science Findings. Download the publication here.
Banner Image: Logs placed in Deer Creek, Willamette National Forest in May 2017 raise the creek bed and connect the river with its floodplain. Photo credit: Sherry Johnson, USDA Forest Service